Until 2004, a blacklegged tick had never been found in Vermont. By 2017, with winters warming, the Green Mountain State led the nation in Lyme disease infections per capita. Re-searchers across UVM work to understand how that happened, and how we can fight Lyme and other diseases that plague humans and other animals.
The first time I saw a blacklegged tick—the primary vector of Lyme disease in the United States—was in 2020, when I noticed one engorged with blood and attached to the base of my toddler’s skull. It was a sickening feeling, and an experience that rarely happened in the Green Mountain State just two decades ago. So, what changed? Seemingly everything, thanks to us.
Lyme disease is caused by spirochetes of the Borrelia burgdorferi bacteria and has been present in North America for over 60,000 years. The bacteria are carried by hoof, paw, and wing across the landscape and were here before humans settled the continent. Only recently have we routinely encountered this disease.
Since the 1960s, blacklegged ticks (and the pathogens they carry) have migrated northward from pockets in the Southeast and along the eastern seaboard as humans segmented the landscape and displaced predators that kept wildlife populations in check. This concentrated where ticks are dispersed. Climate change has worsened the problem, allowing tick populations to overwinter, migrate—and thrive—in new regions.
A Dose of Protection
When Kristen Pierce, principal investigator and faculty director at UVM’s Vaccine Testing Center and a 2003 UVM medical graduate, began practicing medicine in Vermont, her patients never contracted Lyme unless they’d left the state. “Fairly quickly, that changed,” she says.
The first blacklegged tick in Vermont wasn’t discovered until 2004. The first documented case of Lyme disease in the state followed a year later. By 2017, Vermont led the nation in Lyme infections per capita.
“Warmer winters have really changed the geographic distribution, and it’s now in Canada in places like Labrador and Nova Scotia, places that you never see it. … Scandinavia is now endemic,” Pierce says. “And I guess by the same token, there are some places that will climate change out of the distribution because it’s too hot or too dry—but not us, not right now.”
Lyme cases have ballooned since the Centers for Disease Control and Prevention began national surveillance of the disease in 1991, and so has public interest in a potential vaccine. Last fall, the Vaccine Testing Center was inundated with inquiries from people hoping to participate in a phase three trial of a Lyme vaccine developed by Pfizer. There are 9,400 participants enrolled in sites across North America and northern Europe, including 232 individuals in UVM’s arm of the study, which Pierce oversees. The trial tests the vaccine’s efficacy and involves a series of three shots followed by a booster administered this spring.
While attrition is a concern in any clinical trial, Mary Claire Walsh, a nurse and research coordinator at the testing center, suspected it wouldn’t be a problem with the Lyme study.
“Pfizer asked us to complete paperwork with each volunteer to assess their [true] risk of contracting Lyme, and it was a bit comical,” she says. “… If you live in Vermont and you’re walking to the mailbox to check your mail you are at risk for Lyme. So, you can see where the interest comes … and I think it is part of the reason why it’s just so darn important to get a Lyme vaccine here in Vermont.”
The prevalence of Lyme in the region motivated Nina Ridhibhinyo, director of exhibits and education at ECHO, Leahy Center for Lake Champlain, to participate in the study. Not long ago her husband contracted Lyme disease and over the years, Ridhibhinyo has pulled ticks off herself, her toddler, and her pets.
“The constant vigilance is really hard,” she explains, adding that a Lyme vaccine would help reduce the worry of the kids contracting a lifelong illness. “But you still have to manage for ticks. I know other people that have gotten other tick-borne illnesses.”
This is not the first time Ridhibhinyo has participated in a vaccine trial. In her 20s she participated in one for dengue fever because she needed the money. Later, she completed a dengue booster study out of a sense of “scientific duty,” she says. “The Lyme study was a little more out of self-interest.”
But Ridhibhinyo also believes in the work of the Vaccine Testing Center and efforts like it.
“I really believe in the scientific process,” she says, “and I also believe vaccines are important for public health and for individuals.”
UVM’s Vaccine Testing Center was founded by Beth Kirkpatrick, chair of UVM’s Department of Microbiology and Molecular Genetics, and focuses on diseases of global importance. The center’s research portfolio includes vaccines for polio, flaviviruses such Zika, West Nile, and dengue fever—a mosquito-borne illness that poses a risk for nearly half of the world’s population.
“We don’t tend to do large industry-sponsored studies unless we think they have [local] community benefit,” Pierce says.
However, in 2020, the center tested the AstraZeneca Covid vaccine and recruited participants from across New England in the search for an effective treatment.
“It was such a dark time, and I remember when they agreed to let us participate, it felt like this ray of hope—we can do something,” Pierce says. “And I think the same for the Lyme vaccine study.”
The UVM arm of the Lyme study closes in December, after which data analysis can begin to determine its efficacy. However, bringing the vaccine to market requires FDA approval, which can take months—and often years.
“I’m hoping I get the vaccine,” Ridhibhinyo says, referring to the chance she could be part of the control arm of the study. “If not, I’ll just get it when it comes out.”
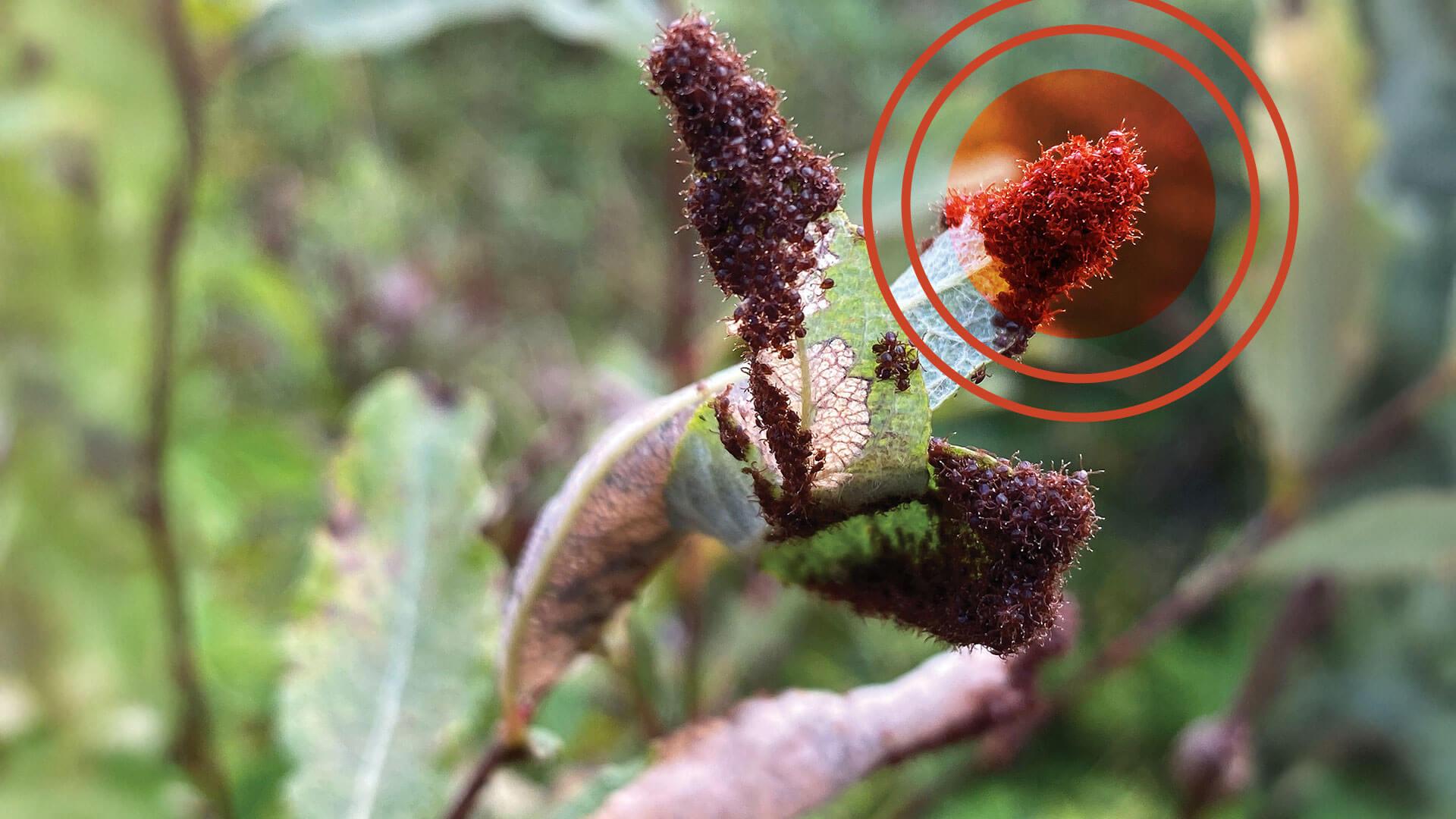
Searching for Spillover
Carlos Amissah is puzzled. The UVM doctoral student in the Department of Biology investigates how ticks, their hosts, and their pathogens are influenced by environmental factors.
“It’s very complex,” he says.
Ticks aren’t born infected with pathogens that cause Lyme disease, anaplasmosis, or babesiosis; they acquire them during blood meals taken over their lifespan. Whether a blacklegged tick becomes a carrier of disease depends on what species they feed upon.
Not all mammals carry pathogens, Amissah explains. “Just the competent ones,” meaning hosts the pathogens can colonize with immune systems they can evade.
In Vermont, the reservoir species for tick-borne pathogens include a diverse, and largely unstudied, set of mammals with different and overlapping habitats. Figuring out which species play a role in disease transmission is further complicated because both blacklegged ticks and their hosts are mobile, with habitat needs that may change throughout their life cycles. For instance, blacklegged nymphs, the first stage of a tick’s life cycle after hatching from an egg, are vulnerable to desiccation and prefer shaded areas or under leaf litter, whereas adult ticks need vegetation to climb when questing for hosts. But even this is not so simple.
“Is the height of the vegetation consistent?” Amissah wonders. “Does native versus nonnative vegetation matter?”
That is just the tick side of the equation. Are pathogens more likely to be present during different seasons?
“I wish I could get a tick to tell you what it’s doing, what the height of a plant is doing, what climate conditions are doing to that tick,” Amissah says. “… There are so many gaps [in tick research].”
He works with Ellen Martinsen ’00, Ph.D. ’09, a parasitologist and head of UVM’s Wildlife Pathogens Lab, to unravel the conditions that make the transmission of pathogens most likely. Amissah’s research involves visiting seven sites in Chittenden County, from farm fields to forest floors, in search of blacklegged ticks. Wearing a permethrin-treated suit and gaiters, he swishes a thick drag cloth across leaf litter and vegetation, checking it every 10 to 15 meters for ticks. Sometimes, he will drag over 100 meters in prime habit and come up empty.
Nymphs tend to cluster, Amissah explains, but a site with robust numbers one summer had only a handful of adults in the fall. Where did they go? Did they attach to hosts? Become food? Was the timing of collection off? These questions churn in his mind.
Tick densities are low since the summer floods, Amissah says. “The numbers are low now, which is not good for my research, but it is good for public health … But low [tick] numbers doesn’t mean low pathogens.”
That is why testing wildlife to see what is lurking inside is critical. Because when the right biological conditions come together it can result in spillover—zoonotic diseases that jump between species—often from wildlife to domesticated animals and to people. And that is happening now.
In mid-January, when Martinsen presented preliminary findings from her team’s tick research to UVM graduate students and faculty members, the H5N1 avian flu strain, first detected in the United States in 2022, had infected more than 900 American dairy herds and domestic cats, and caused the loss of more than 138 million birds across the country.
“I don’t need to convince anyone that we are living in an age of emerging and re-emerging diseases,” Martinsen tells the audience.
She points to a slide showing diseases that spilled over from wildlife to wreak havoc on human health including malaria, HIV, monkey pox, EEE, Chagas, and SARS-CoV2. It works the other direction too—pathogens we unknowingly collect and trek across the globe can harm wildlife. For instance, since 2006, white-nose syndrome, likely transmitted by human foot traffic, has decimated domestic bat populations, which are voracious eaters of insects and potentially reduce insecticide use by farmers.
In recent years Martinsen’s lab has pivoted from studying malaria parasites in wildlife species to tick-borne pathogens and small mammals.
“Ticks are responsible for 95 percent of vector-borne illnesses in the United States,” she says. “Ticks have become a plague across the United States and across the world. … It really is a One Health issue as well as a planetary health issue.”
Martinsen aims to determine which small mammal species in Vermont are hosts for pathogens that can cause severe illness in humans including Lyme, anaplasmosis, and babesiosis. While Lyme is the most common pathogen transmitted by blacklegged ticks, about 5 percent of ticks test positive for all three and it remains unclear how these pathogens affect each other. Does having one pathogen make a tick more likely to contract another?
Martinsen tested coinfection dynamics to find out. Using both adult and nymph populations of blacklegged ticks collected in Chittend-en County, her team found that adults were nearly twice as likely to carry Borrelia burgdorferi. However, the same percentage of nymphs and adults had Anaplasma phagocytophilum and Babesia (9 and 4 percent, respectively), indicating they pick up those infections as nymphs.
And if a tick has Babesia, they are way more likely to have Anaplasma, Martinsen says. “We found co-infections in all seven sites. We even found ticks that have all three infections.”
Curiously, of the 147 white-footed mice her team examined—a known reservoir for Borrelia burgdorferi—55 percent tested positive for it, but none had Babesia or Anaplasma, suggesting that ticks are picking up those pathogens from other hosts. But from which ones?
Martinsen’s team of undergraduate and graduate students have turned to small mammals to find out. To test for pathogens, she read-ily collects roadkill and utilizes small mammal “bycatch” from the Vermont Reptile and Amphibian Atlas’ studies, which sometimes find drowned mice, voles, shrews, and moles inside their watery traps. These samples are provided to UVM’s Natural History Museum, where interns prepare them as specimens for the collection and collect tissue samples. From this collaborative work new culprits have surfaced. The meadow jumping mouse had all three pathogens present. Woodland voles tested positive for them, too. Same with northern short-tailed shrews.
Many of these species are under-represented in tick-borne pathogen studies and therefore as potential reservoir hosts for pathogens in part because of how we trap small mammals and bait traps, Martinsen explains. When traps use peanut butter or seeds, it inevitably attracts the species that eat those foods and not the insectivores. Sometimes we miss what we aren’t looking for.
“The system is so stupidly complex,” she says. “We are really lucky that some pieces we found fit together to tell a story … There is still so much work to be done.”
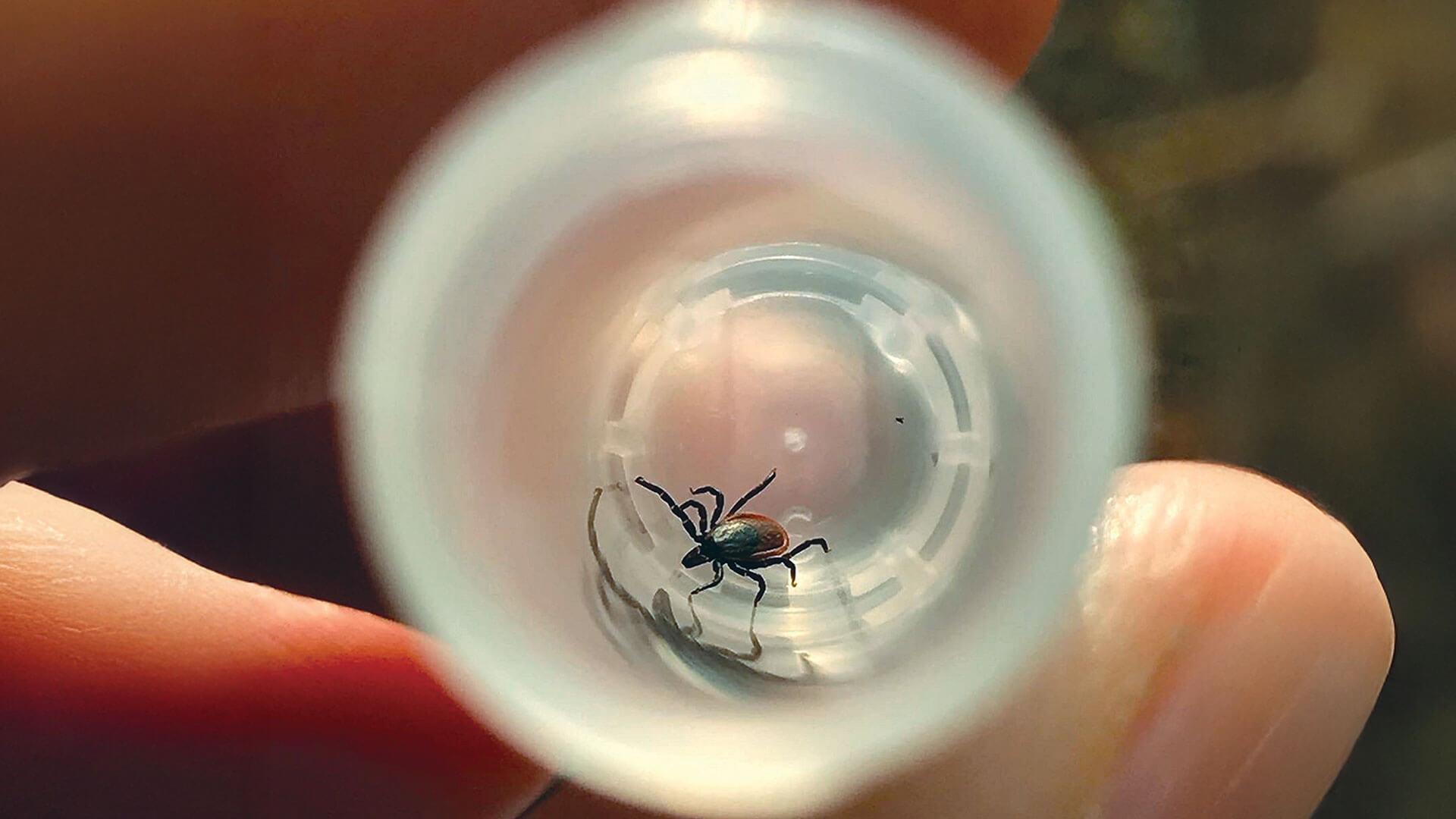
Vector, Meet Host
Cheryl Sullivan ’03, ’12, Ph.D. ’21 peers into a microscope, tuning a knob to bring the contents of a clear container into focus. She gestures for me to look. A brown tick, nearly translucent, with lavender markings and wisplike hooks on each leg, crawls into view. With-out magnification, the month-old blacklegged tick larva resembles a graham cracker crumb.
“They are very soft when they hatch,” says Sullivan, an entomologist in the Department of Agriculture, Landscape, and Environment and member of UVM’s Entomology Research Laboratory. “It takes a few days for their exoskeleton to harden.”
Sullivan studies entomopathogenic fungi—fungus that cause disease in insects and other arthropods, like mites and ticks—and tests their efficacy as biological control measures for pests. Some species are found in soils around the world, including Vermont, and have
evolved to exploit arthropods. The fungi produce spores that germinate and penetrate arthropod exoskeletons and slowly kill the ticks from the inside.
They can be quite effective, Sullivan says, but the fungi take longer to kill ticks than a chemical pesticide that doesn’t distinguish a pest from a beneficial insect. Timing is key.
“You have to really get them at the right stage of their life to be effective,” she says. “For me, targeting the larval stage is prime time.”
That is when ticks are most vulnerable to environmental conditions and have a greater chance of encountering fungi on the forest floor. There is only one fungal biopesticide available for ticks in the United States and limited for residential use. Part of Sullivan’s research involves hunting for more biocontrol agents. She collects samples of Metarhizium found in Vermont soils and develops a solution from the isolated spores to see how they perform against ticks. The arthropods are rinsed with the mixture and observed for mortality.
“Fungi are very widely used against pests in agricultural production and forestry,” Sullivan says. “But for ticks, for some reason, it has been kind of slow to catch on.” At least, in the United States. In parts of the world where cattle ticks have developed insecticide resistance, fungi are frequently deployed. The time and expense of conducting research on ticks may be prohibitive from developing new fungal products, too. Ticks are not like fruit flies, with incredibly short lifespans—some tick species take years to complete their life cycle.
“It takes years of research to demonstrate things actually work,” Sullivan explains.
She swaps containers and readjusts the microscope to show the results after a biopesticide application. The remains of 10 larvae are mostly just clusters of spores and some legs.
Many of the ticks Sullivan tests are collected from the landscape or shared from state surveillance programs and big game check stations run by the Vermont Fish and Wildlife Department that collects biological data to inform their management plans. These efforts feed into a regional One-Health approach to tick surveillance and management launched last year with researchers from the University of New Hampshire and University of Maine. The aim is to establish long-term surveillance sites and identify emerging threats to hu-mans and wildlife.
Tick surveillance has to be done in an all-hands-on endeavor, Sullivan says. “Right now, … it’s funded primarily by the CDC and run by the state departments of health, and they only have so much funding. … We should all be working together collectively to forecast tick-borne diseases and get more of a baseline as to what ticks and pathogens are circulating. A lot of times we are going to find those in wildlife before in humans—hopefully.”
In 2018, New Hampshire documented the arrival of the Asian longhorn tick on a dog visiting from out of state. They are an invasive species that reproduces by cloning and quests for hosts in clusters. Sullivan is also watching for the lone star tick, which has been found in growing numbers in parts of Maine.
“Who knows what other sort of ticks we might find?” she says. “Not all ticks like to hang out on the ecotone. Some like fields. Some like different habitats that we are just not checking.”
New ticks may be found with additional surveillance, but not all ticks pose threats to health. There are 15 known species of ticks in Vermont, but only about half cause illness in humans. Vermont’s Agency of Agriculture Food and Markets and Department of Health conduct annual surveillance for five disease-causing pathogens—the big three—as well as hard tick relapsing fever and Powassan virus, a rare but potentially fatal illness.
“Ticks are classified as being either one-, two-, or three-host ticks, and it depends on how many hosts that they use throughout their life cycle,” Sullivan says. “It really is like an apple and orange.”
Winter ticks are one-host ticks that typically complete their life cycle on a moose. In the fall, larvae latch onto moose and overwinter under a protective thick coat of fur with adult females taking a final blood meal in the spring before dropping off to lay eggs. Black-legged ticks switch hosts between blood meals, increasing their chances of collecting and passing pathogens to other animals (and humans). Climate change has pushed frost dates later in the fall and thaws earlier in the spring, giving ticks more time to quest for hosts and hatch in ideal conditions. It’s a numbers game—and the ticks are winning.
“That is why you are starting to see more cases [of tick-borne illnesses] popping up in wintertime, because people are getting bites all the time,” Sullivan explains. “If it is above freezing, and especially if there is no snow cover, those ticks are going to be active.”
Sullivan herself was bitten by a tick last January.
“Me of all people!” she says.
“There is no one silver bullet to tick control,” Sullivan admits. “Integrated pest management is a collection of strategies that work together, and a lot of these strategies … focus on detection and personal prevention. Because first and foremost, tick bites are preventable.”
A Deadly Problem
“I could talk for hours about moose,” says Joshua Blouin, ’15, M.S. ’21, a wildlife biologist at Vermont Fish and Wildlife, with a smile.
At UVM, he studied how winter ticks affect the health and mortality of Vermont’s moose population to understand the factors influencing moose survival in the Northeast. In 2017, Blouin and a team of researchers began tracking calves and female moose in Essex County, where the ungulate population is the highest—and most vulnerable—to succumbing to winter ticks. The first and most crucial data point collected was the animal’s live weight.
“That live weight was pretty telling,” Blouin explains. “If we collared a calf in January and it was already fairly small, then it’s chances of withstanding blood loss from winter ticks is pretty slim.”
Moose calves are typically born in May and may weigh about 350 pounds by January. If a calf survives its first 60 days, its survival rate goes up exponentially, Blouin says. “Pretty much nothing messes with a full-sized moose.”
At least, this used to be the case. By April, moose calves can lose a third of their body weight as they lose conditioning over winter and sustain severe blood loss from ticks. During epizootic years when calf mortality is greater than 50 percent, the winter tick load is al-most unfathomable. Blouin’s team estimated one calf in the study had 70,000 ticks embedded in its skin. In New Hampshire, some moose have been found with over 90,000 ticks.
“A lot of people think that moose are dying from a disease related to ticks, something like Lyme disease,” Blouin says. “Because most people’s interactions with ticks is human-related health. … But that is not what’s happening with moose.”
They are dying slow anemic deaths because they can’t replenish their blood fast enough. Winter tick nymphs, the size of pepper flakes, cluster by the thousands on the tips of vegetation in the fall and wait until an animal—ideally a moose—passes by if a hard freeze or snow doesn’t come first. When filled with blood, they swell to the size of small grapes.
“Most people think a tick is a tick in Vermont,” Blouin says.
But winter ticks are specialists. They likely evolved with moose since other species such as deer and bear tend to groom them off.
“Moose don’t,” Blouin explains. “Moose are really their primary host—that is what drives that winter tick population.”
Winter ticks don’t move far on their own; they go where moose go. And ideal moose habitat is a patchwork of older forests that pro-vide cover in warmer months and younger forests offering forage. Using GPS data from calves and their mothers, Blouin fleshed out the geography of the animal’s first year of life. A telling pattern emerged. The survivors weren’t often congregating in high-trafficked areas—“in areas where we would expect moose to be,” Blouin says. “They were kind of on the fringe of optimal moose habitat.”
More moose typically means more winter ticks. But how many are too many? And what, if anything, should we do about them? Unlike blacklegged ticks, winter ticks are not new to Vermont. And scientists are uncertain what their role is in the ecosystem.
“They have had an interaction and a coexistence with moose for a long time as far as we know, which makes that complicated as well in terms of how we’re dealing with it,” Blouin says.
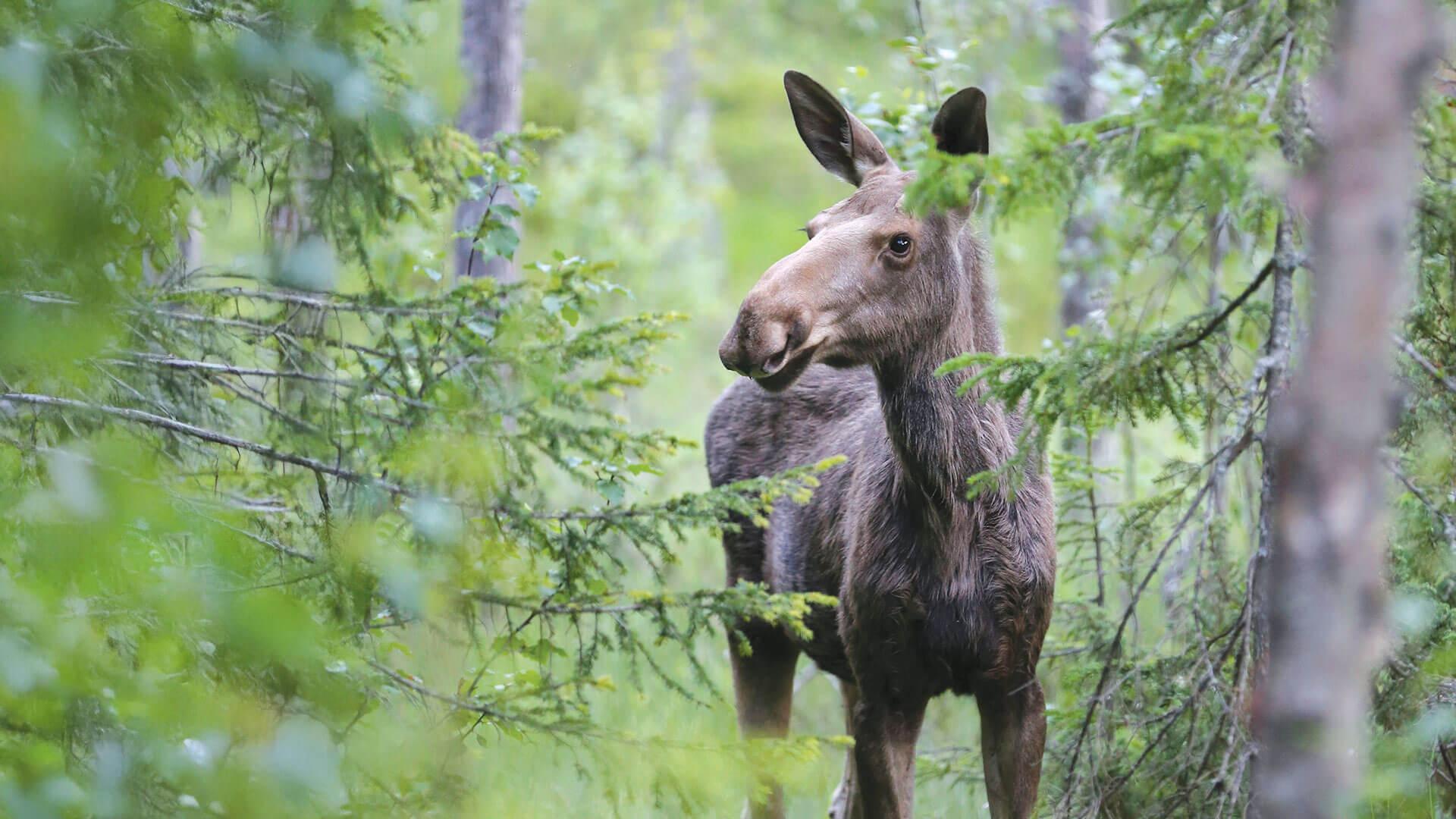
Cycles of Change
The story of Vermont’s moose is one of fluctuation. During the 1800s, sheep grazing deforested much of the state, pushing moose out of the region. Predators including mountain lions and wolves (and hunters) kept the moose population in check before that. As Vermont reforested, moose returned to a landscape without apex predators and flourished in their absence.
“We went from 25 individuals in the 1960s to 5,000 individuals in the early 2000s,” Blouin says. “So, we know moose populations are able to rebound quickly.”
And as moose have thrived, winter ticks have too. Now, their populations are regulating moose numbers—but it’s a long and painful process.
“It might take 30 years,” Blouin says. “Because we are talking about densities of moose that were over five per square mile. … A normal moose density across North America is less than one moose per square mile, so, our numbers of moose were way too high.”
People often ask why we don’t spray the forests with insecticides or equip moose with tick collars, Blouin says. “At this point in time, it’s not feasible, and that is for a variety of reasons.”
Not only would it be expensive to target moose calves each winter, but these interventions could have unknown repercussions on water quality or on scavengers like fox and bald eagles.
“The moose winter coat is thick. Would a flea collar even work?” he asks. “And at what dose?”
Cheryl Sullivan’s research on biopesticides is intriguing, he continues, but far from implementation in forests. And unlike the Lyme vaccine for humans, there is not one for winter ticks in development. That leaves wildlife managers with few options. Since moose numbers drive winter tick infestations, wildlife agencies across the Northeast harvest moose to reduce densities of both species and return to a healthier baseline.
Vermont’s moose population has stabilized but remains greater than one per square mile, Blouin says. “That is kind of the magic number.”
In the meantime, the department continues to gather health data over camera traps and during the fall harvest, including gathering ticks for Sullivan’s research.
“I’ve really enjoyed working on the moose project because I love moose,” Blouin says. “But I also enjoy working with moose because people care. … People want to see them on the landscape, and people want them healthy.”