There are no fruit flies in Davi Bock’s kitchen. But head down into the basement of his 1850s farmhouse, on the end of a dirt road in Glover, Vt., and you’ll find them by the millions. Well, not really. But—in one corner, on a laptop computer linked to the wider world with high-speed, fiber-optic cable—you can surf and plunge into 21 million photos of one fruit fly’s brain.
Bock points to a spectral gray image, roughly the shape of Princess Leia’s hairdo. “This is the optic lobe,” he says, pointing to one side of the picture that sticks out like a bun. “You’re seeing hundreds of images mosaic together to encompass the whole fly brain from tip to tip.” On a large monitor, the brain appears about a foot wide. In an actual fruit fly, the brain is smaller than a poppy seed.
Inside that speck, some 140,000 neurons are linked together—hundreds of feet of microscopic, living spaghetti—to form more than 50 million connections called synapses. Bock—a biologist who grew up in Jericho, Vt., and joined the faculty of UVM’s Larner College of Medicine in 2019—co-led an effort, over the last 12 years, to photograph, trace, and label every neuron and synapse in a fruit fly’s tiny head. His work, which yielded the first complete map of the neural wiring in the brain of a complex organism, made the cover of the journal Nature in October 2024—and was reported by news outlets around the world, including the BBC, which described it as a “huge leap to unlock the human mind.”
“Now we’re going to zoom in,” Bock says, leaning forward with the hint of a smile as his finger moves over the trackpad. The gray image grows larger and soon we’re plummeting down toward the surface of some monochromatic planet and plunging below the surface. We’re passing through the fruit fly brain from front to back as the scale grows finer. “This is the esophagus,” Bock says, pointing to a wedge-shaped hole in the center of the image, “it passes through the fly’s brain.” Rushing ever deeper into the stream of images is dizzying and topographic. Another Star Wars image presents—I found myself thinking about Luke Skywalker roaring through an abstract canyon on the Death Star, except we’re flying through layers of photos of a real fly in the real world.
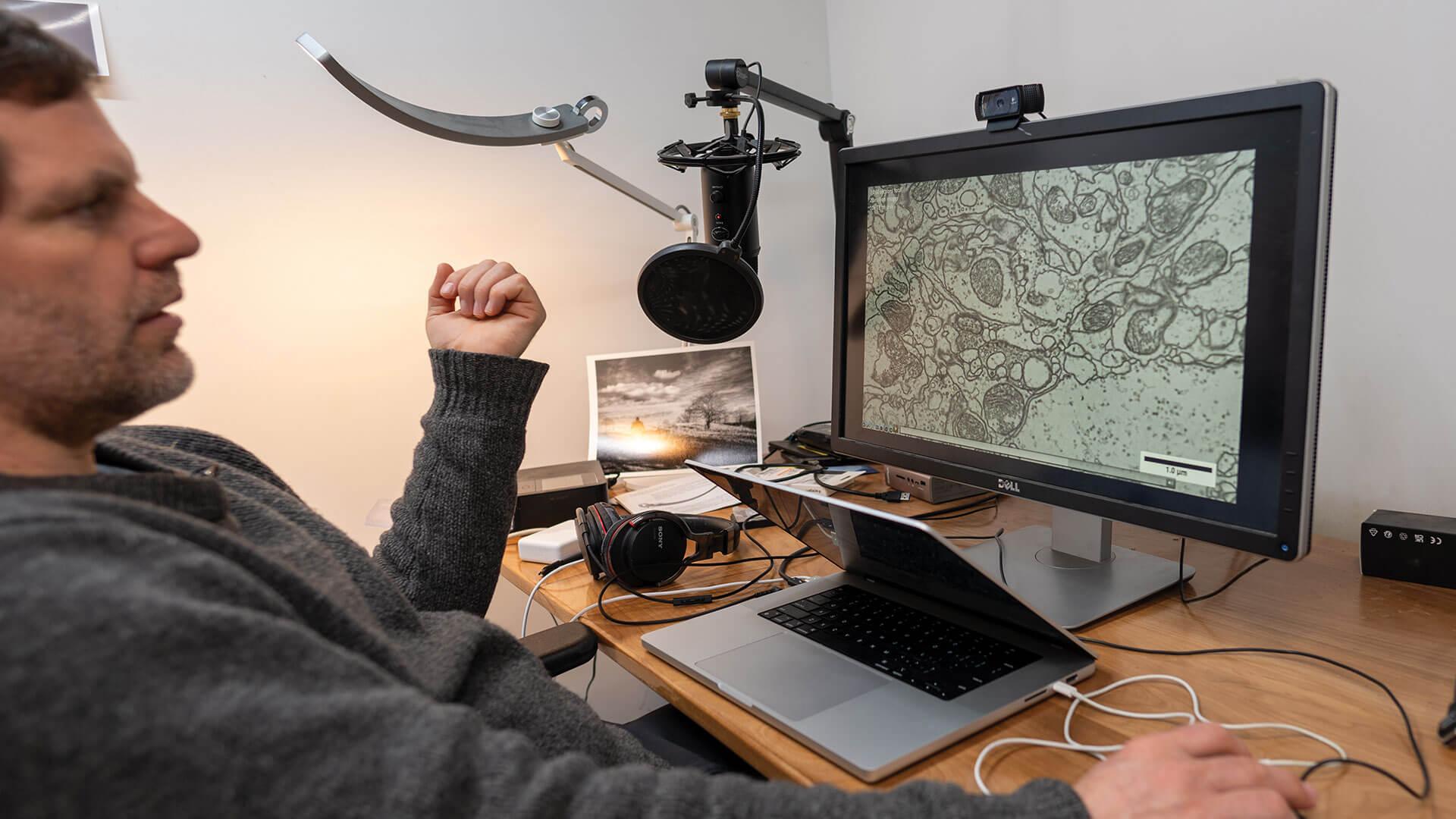
“Now you can start to see individual cell bodies. These are called tracheae; they’re tubes that supply the fly’s brain with air,” he says, pointing to what looks like black-and-white salami. Soon we reach the finest scale—the whole screen now shows just a single micrometer, a hundred times smaller than the diameter of a human hair. We are deep within a single brain cell. “These are individual synaptic vesicles, forty-nanometer-diameter spheres containing neurotransmitter,” he says pointing to bead-shaped dots. “Oh, these are mitochondria, the energy sources of the cell,” he says, pointing to some darker, bean-shaped masses. “And this is a synapse,” he says, pointing to where the cell forms a link, connecting this neuron to another, an infinitesimal gap where molecules of neurotransmitter jump, carrying a packet of information. And these bits of chemical information—launched by electrical signals that move up and down different types of neurons, each one splitting into a starburst of microscopic threads that connect with many other neurons, forming bunches and bundles and long highways, all gathering in layers and knots of increasing complexity—result in a fruit fly that can sing to attract a mate.
Yes, fruit flies sing. Those pesky critters that drown themselves in your glass of wine, or swarm on your bananas, have complex courtship behaviors. The males serenade females, choosing between songs—distinctive vibrations with their wings—and deciding in the moment when the best moment for romance has arrived. (Patience, young fruit fly, females take fully 8 to 10 hours after hatching to become sexually mature.) “You might think, ‘fruit fly, whatever; it’s just this little dot floating around your compost,’ but actually they’re pretty sophisticated. They do this thing called ‘visual place learning,’” says Bock, leaning back in his chair. If flies are put in a hot cage with visual cues showing one cool tile that’s comfortable to stand on, they form and retain memories of where to run to, following the cues when they’re moved. “They fight, they learn, they forget,” Bock says.
In other words, fruit flies are not that different than people. They can get drunk, figure out how to navigate from one point to another, get jacked on coffee, and respond to dark and light—fruit fly research led to the discovery of circadian rhythms, the 24-hour cycle that regulates sleep patterns, hormone release, and body temperature. We share many of the same genes—75 percent of the genes involved in human diseases are also found in fruit flies, including two-thirds of human cancer genes. When scientists insert a human gene associated with Parkinson’s disease into fruit flies, they display symptoms like those seen in humans with the disorder.
Those similarities are one of the reasons that thousands of researchers dig into the inner workings of these tiny creatures. A so-called model organism (along with E. coli bacteria, laboratory mice and rats, zebrafish, and a few others), the biology of the fruit fly, Drosophila melanogaster, has been studied intensely for more than a century, revealing principles of physiology, development, genetics, diseases, and evolution. It was the first animal to be launched into space, in 1947. And today, hundreds of lines of medical research rely on fruit flies—including efforts to understand and treat diabetes, neurological disorders like Huntington’s disease, drug addiction, eating disorders, and cancers. The newly completed brain map—what scientists call a connectome—is helping to advance understanding of how pathways in the brain link to diseases and behaviors.
Bock clicks on the computer and the trip continues through the millions of stitched-together photos, zooming back out to the composite image of the whole brain and then diving down into another area. “I remember when I got the software put together to view images of this scale—I believe I was the first person in human history to be able to do this,” he says, “to go from the resolution of individual synapses to seeing all layers of the brain’s cortex in a single image.”
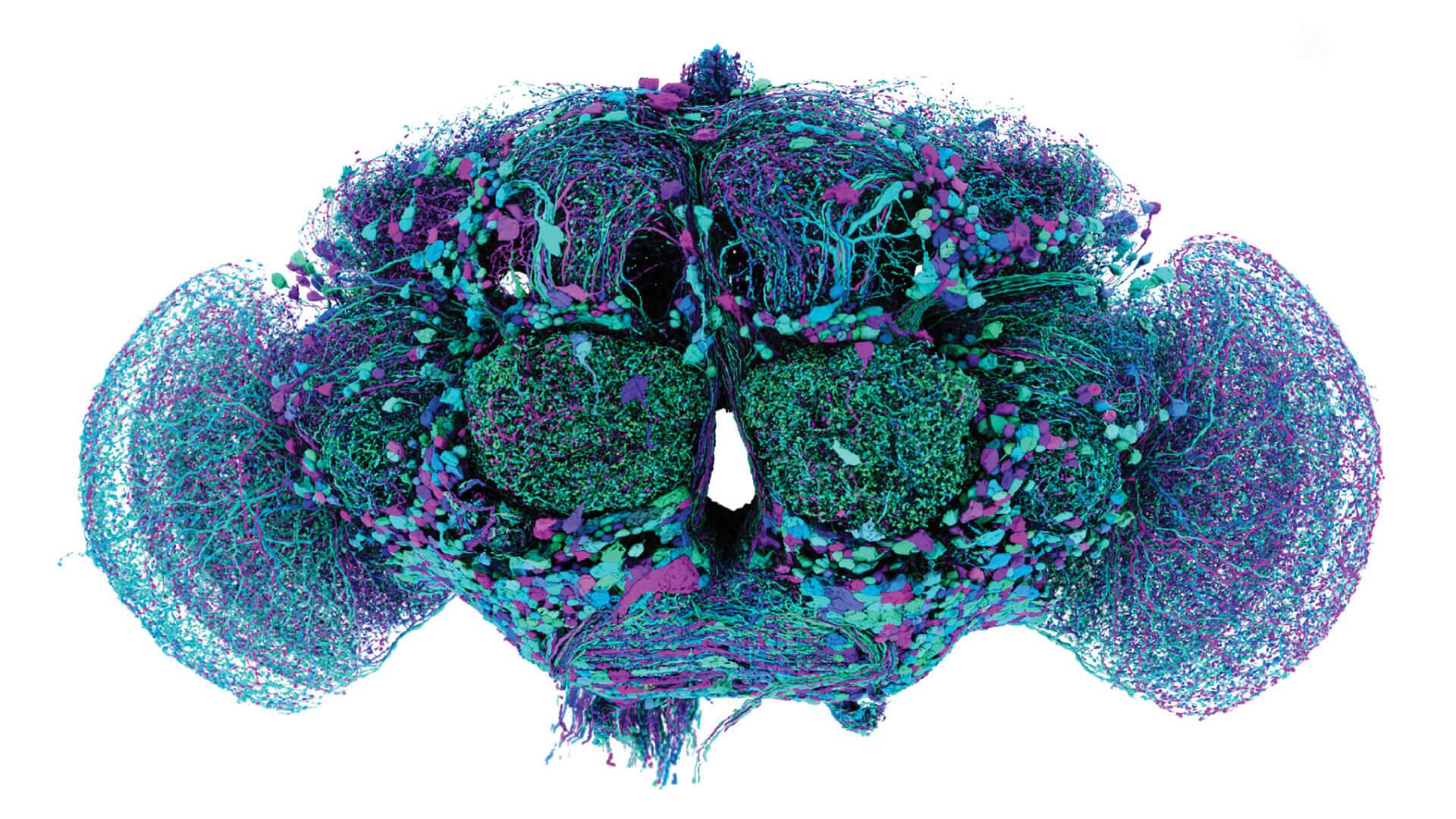
Bock studied biology, and taught himself computer programming, at Brown University, graduating in 1997. “I love biology, but I had a really hard time in college. I just hated—and I still hate—being told what to do with my mind,” he says. “You lock in on a class and you have a syllabus and there’s a schedule, and you have to learn this by then and so on.” After college, he went to work as a software consultant, made some money in the dotcom boom, and, a few years later, returned to Vermont, where he “reconnected with old friends, played D&D, went to life drawing classes, learned some banjo, and basically screwed around,” Bock recalls. “But then, of course, you get tired of screwing around and the savings starts to run down.” So, in a fateful decision, he went to work as a technician in the UVM laboratory of neurobiologist Rae Nishi. A beloved professor, Bock credits her with giving him the support and confidence to pursue a research career of his own. “I started out washing glassware and making stock reagents and placing orders, but she gave me a project and taught me skills—and I started working day and night,” Bock says. He reluctantly left Vermont in 2007 to start a Ph.D. at Harvard, where he outfitted a custom high-throughput electron microscope that let him gather microscopic images of portions of a mouse’s brain at a scale never before achieved. This work was published on the cover of Nature in 2011. “I got to see the brain in a way that nobody else ever had,” he says. “It was like landing on the moon.”
The mapping of the whole fruit fly brain began in 2013. Bock, then a scientist at the Janelia Research Campus of the Howard Hughes Medical Institute in Virginia, and his colleagues extracted the brain of an adult fly and dipped it into a bath of heavy metals and other chemicals, allowing it to harden. Once they had a solid block, they cut it with a diamond knife into 7,062 ultra-thin slices. Then they used a pair of custom electron microscopes with robotic loaders and high-speed cameras—created by Bock with help from a few consultants and Janelia staff—to scan each slice, producing 21 million pictures. In these images, each pixel represents “a crazy level of resolution,” Bock says: a bit of brain tissue no wider than a few dozen atoms. This first-of-its-kind snapshot of an adult fruit fly brain was published in 2018 in the journal Cell.
But years of hard work lay ahead to transform Bock’s photographs into a digital model. That work was completed by a project called the FlyWire Consortium—an unlikely global effort of hundreds of neuroscientists, citizen volunteers, gamers, and professional tracers—housed at Princeton University and led by two professors there, Bock’s collaborators Sebastian Seung and Mala Murthy. Using an AI system and other algorithms, the blobs and lumps in the images were turned into a rough-draft, three-dimensional map. Then human proofreaders painstakingly went through the map, correcting and refining it, checking every synapse, annotating every neuron, and finding others that the computers had missed. Along the way, Bock—with his close collaborator Greg Jefferis at the MRC Laboratory of Molecular Biology and University of Cambridge, and other scientists—identified more than 8,400 different types of neurons in the fly’s brain.
With map in hand, researchers around the world are now exploring how the wiring in the brain connects to behaviors and diseases. “It’s turned out to be helpful to understanding how the fruit fly processes sensory information,” Bock says, and then he calls up the 3-D model of the entire fly connectome on the screen. The black-and-white images are swept away by a gorgeous, rotating tangle of multicolored threads and neon masses. It looks like Princess Leia’s hairdo on LSD. In the special issue of Nature where this new map was published, one team of researchers describe a computer model they built testing how the fly brain interacts with sensory inputs—based on the map. “Detection of appetitive substances,” they write, “results in proboscis extension.” In other words, when given a tasty treat, a brain circuit in their simulated (and hungry) fly sends a signal to stick out its tongue. The map works.
I’m eating lunch with Davi Bock in the cafeteria of Given Building at UVM’s Larner College of Medicine, interviewing him over tacos, while a slow stream of surgeons and medical students pick up sandwiches to go. He’s a research professor here, in the Department of Neurological Sciences, but spends most of his time at home, on 192 acres of mixed forest and meadow in the Northeast Kingdom, where he plants apple trees and skis to his woodlot when he’s not on his computer. “I’m really not a conventional academic scientist, for better and for worse,” he says. “I grew up in Vermont on a dirt road with five farms, all of them failing. The Eighties were not a kind time for agriculture of that scale, but I fell in love with it. I was imprinted by that landscape and the natural world resonated. I have what E.O. Wilson calls ‘biophilia’—a deep love of nature. I have a severe case of biophilia, arguably crippling.”
So—when Bock left the world-renowned Janalia Research Campus, leading a large laboratory group outside of Washington, D.C.—he decided to come home to Vermont with his wife and two children. “I just don’t want to live in cities ever again,” he says, “and UVM welcomed me back.” The interview is almost over and he wants to know if I have any more questions, so I slide my list over the table to him and point to this one: “Will you ever be able to upload your brain?” He doesn’t say a word, but just pulls out a black felt-tip pen and writes, in all-caps, “NO.”
The connectome of the fruit fly is complicated compared to the previous biggest brain map of an adult animal—a roundworm called C. elegans which has a Homer Simpson-worthy 302 neurons. The brain of the fruit fly is many orders of magnitude more complex and moves scientific understanding of neurobiology closer to a long-imagined goal: a complete map of the neurons in the human brain.
“But the problem is that our brains are too big, and so we start with the fruit fly,” Bock says. “The whole-brain connectome of a fruit fly is a demonstration of how circuits work at a basic level and reveals patterns across different kinds of brains. Take epilepsy. How does a focal seizure—that starts at one spot in the brain—propagate and kindle activity brain-wide? These kinds of questions are deeply informed by wiring diagrams. It’s like you have a new kind of flashlight and can point it around at whatever you like.”
Illuminating the human connectome is going to take a mighty bright flashlight. If fruit flies are a lot like people in some ways, they are also deeply, maybe radically, different too. For one thing, they’re a lot better at flying than we are. But it also seems safe to assume that we’re a lot better at thinking and reasoning and wondering about how we think. At the foundation of this difference is the profound reality that a fruit fly has millions of connections in its brain while the human brain may be the most complex structure in the universe. “You can estimate how many neurons there are, and it’s roughly a hundred billion,” says Bock. Inside your head, these neurons create some hundred trillion synapses, far more than all the stars in the Milky Way—a fact, discovered by human brains, that you can contemplate while looking up into a dark night sky, aware of your awareness.
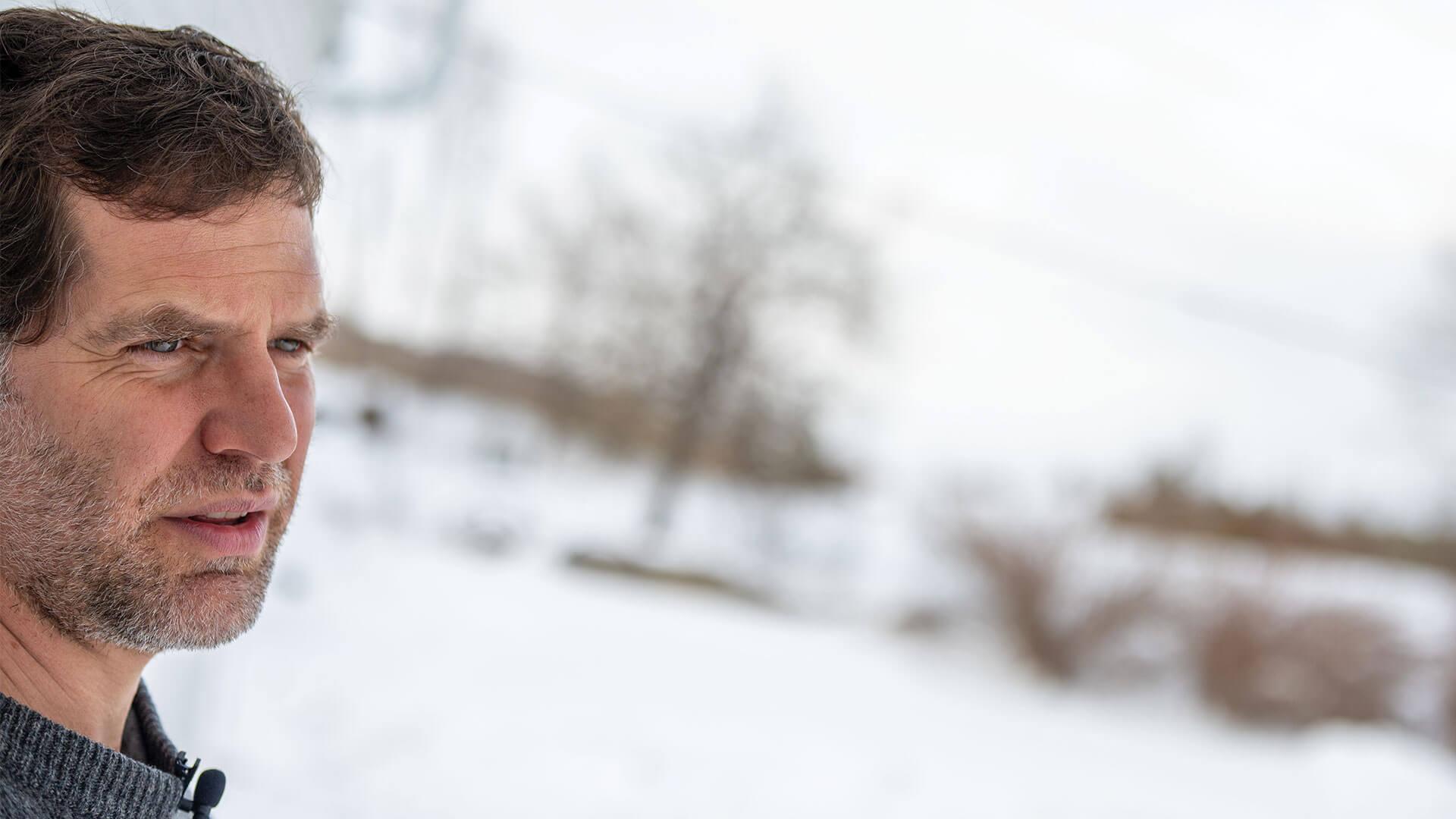
“You know, for a while, I was becoming a neuro-nihilist. I spent 20 years trying to build these wiring diagrams—and began to wonder whether they would really help us understand,” Bock says, “but I have some cause for optimism recently, and it’s really motivated by these integrative simulations that are starting to happen.” One of Bock’s colleagues at Janelia used the wiring diagram to model part of a fly’s eye and the simulation reconstituted what the cells do in a living animal. “The simulations seem to capture the essence of what brain circuits do. And, importantly, that didn’t work without the connectome, without the map.”
Bock’s colleague at Princeton, Sebastian Seung, has a TED talk in which he asks the audience to chant, “I am my connectome.” Bock is not so sure. If his work on uncovering the wiring of the fly brain has promise in helping us understand some human behaviors and diseases, it’s not because the full explanation of identity or consciousness comes from a brain map, no matter how intricate. If you want to explore New York City, a map sure helps. And walking First Avenue, checking out Google Maps is great for finding any building, subway entrance, or juice bar you need to find. But even a map that identified every person, taxicab, smell, and pigeon flying by—would not be a full explanation of the city. The same is true of a connectome. The hundred trillion synapses in your brain are but the scaffolding for a vastly more complex set of interactions in which the strength of electrical signals, the amount of neurotransmitter, the inputs from the bloodstream, the quarkish patterns of information at the atomic scale, are a constantly shifting cascade. The map is not the territory.
“Consciousness arises from matter. There’s just a profound mystery there. Matter has organized—seven billion times on the planet right now—to become aware of itself. That’s just bizarre,” Bock says. Most people agree that our brains are the basis for our consciousness, and they’re how we can perceive and remember and control our bodies, and they’re part of where our emotions come from. “So it’s natural to wonder how our brains work the same way we wonder how our heart works or how our skeletal system works,” Bock says. “If you don’t believe in supernatural causes for consciousness, you start to wonder, well, how did it happen?”
Bock pushes his chair back and gathers his bag to leave. “What fraction of the explanation is the wiring diagram? And the answer is a small fraction,” he says with a rueful laugh—then he makes himself stop laughing. He fell down skiing and broke a rib and laughing hurts. His brain senses pain, so his brain stops him from laughing. Or his brain creates pain, so he uses his brain to stop laughing. Or something. “We may not understand this stuff yet, but there seems to be a path to getting there. And that path is, I think, greatly facilitated by having these wiring diagrams. So that’s reduced my neuro-nihilism.”
“The fact is that even with these wiring diagrams, consciousness is still a mystery, but at least we’re closer to it, sitting next to it,” he says. “You’re sort of consciousness-adjacent. You start to get a sense of how it might work, even if you don’t really know.”
As I head out of the building, I think about going back in to ask him more about uploading our brains. In our conversation, he said that, even if we could, he wouldn’t want to, afraid that the digital copy of his brain would be low-fidelity. “It’s very likely to be some weird, altered thing that just doesn’t have much to do with me,” he said. I want to ask him if he’d like to make a copy of his brain if he knew that it would be a perfect copy—but then I think the better of it and keep walking out into the sunshine. His reasoning seems clear, and he’s made up his mind.